图书介绍
GAS TURBINE HEAT TRANSFER AND COOLING TECHNOLOGY【2025|PDF下载-Epub版本|mobi电子书|kindle百度云盘下载】
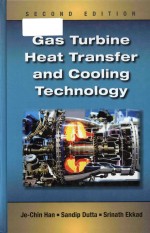
- SECOND EDITION 著
- 出版社: CRC Press;Taylor & Francis
- ISBN:9781439855683
- 出版时间:2013
- 标注页数:869页
- 文件大小:313MB
- 文件页数:886页
- 主题词:
PDF下载
下载说明
GAS TURBINE HEAT TRANSFER AND COOLING TECHNOLOGYPDF格式电子书版下载
下载的文件为RAR压缩包。需要使用解压软件进行解压得到PDF格式图书。建议使用BT下载工具Free Download Manager进行下载,简称FDM(免费,没有广告,支持多平台)。本站资源全部打包为BT种子。所以需要使用专业的BT下载软件进行下载。如BitComet qBittorrent uTorrent等BT下载工具。迅雷目前由于本站不是热门资源。不推荐使用!后期资源热门了。安装了迅雷也可以迅雷进行下载!
(文件页数 要大于 标注页数,上中下等多册电子书除外)
注意:本站所有压缩包均有解压码: 点击下载压缩包解压工具
图书目录
1.Fundamentals1
1.1 Need for Turbine Blade Cooling1
1.1.1 Recent Development in Aircraft Engines1
1.1.2 Recent Development in Land-Based Gas Turbines3
1.2 Turbine-Cooling Technology5
1.2.1 Concept of Turbine Blade Cooling5
1.2.2 Typical Turbine-Cooling System7
1.3 Turbine Heat Transfer and Cooling Issues14
1.3.1 Turbine Blade Heat Transfer14
1.3.2 Turbine Blade Internal Cooling18
1.3.3 Turbine Blade Film Cooling21
1.3.4 Thermal Barrier Coating and Heat Transfer21
1.4 Structure of the Book22
1.5 Review Articles and Book Chapters on Turbine Cooling and Heat Transfer23
1.6 New Information from 2000 to 201024
1.6.1 ASME Turbo Expo Conference CDs25
1.6.2 Book Chapters and Review Articles25
1.6.3 Structure of the Revised Book26
References26
2.Turbine Heat Transfer31
2.1 Introduction31
2.1.1 Combustor Outlet Velocity and Temperature Profiles31
2.2 Turbine-Stage Heat Transfer35
2.2.1 Introduction35
2.2.2 Real Engine Turbine Stage35
2.2.3 Simulated Turbine Stage43
2.2.4 Time-Resolved Heat-Transfer Measurements on a Rotor Blade49
2.3 Cascade Vane Heat-Transfer Experiments52
2.3.1 Introduction52
2.3.2 Effect of Exit Mach Number and Reynolds Number53
2.3.3 Effect of Free-Stream Turbulence57
2.3.4 Effect of Surface Roughness58
2.3.5 Annular Cascade Vane Heat Transfer62
2.4 Cascade Blade Heat Transfer66
2.4.1 Introduction66
2.4.2 Unsteady Wake-Simulation Experiments67
2.4.3 Wake-Affected Heat-Transfer Predictions74
2.4.4 Combined Effects of Unsteady Wake and Free-Stream Turbulence78
2.5 Airfoil Endwall Heat Transfer83
2.5.1 Introduction83
2.5.2 Description of the Flow Field83
2.5.3 Endwall Heat Transfer86
2.5.4 Near-Endwall Heat Transfer88
2.5.5 Engine Condition Experiments90
2.5.6 Effect of Surface Roughness92
2.6 Turbine Rotor Blade Tip Heat Transfer94
2.6.1 Introduction94
2.6.2 Blade Tip Region Flow Field and Heat Transfer95
2.6.3 Flat-Blade Tip Heat Transfer98
2.6.4 Squealer- or Grooved-Blade-Tip Heat Transfer99
2.7 Leading-Edge Region Heat Transfer106
2.7.1 Introduction106
2.7.2 Effect of Free-Stream Turbulence108
2.7.3 Effect of Leading-Edge Shape113
2.7.4 Effect of Unsteady Wake114
2.8 Flat-Surface Heat Transfer118
2.8.1 Introduction118
2.8.2 Effect of Free-Stream Turbulence118
2.8.3 Effect of Pressure Gradient123
2.8.4 Effect of Streamwise Curvature124
2.8.5 Surface Roughness Effects126
2.9 New Information from 2000 to 2010128
2.9.1 Endwall Heat Transfer128
2.9.1.1 Endwall Contouring128
2.9.1.2 Leading-Edge Modifications to Reduce Secondary Flows130
2.9.1.3 Endwall Heat-Transfer Measurements131
2.9.2 Turbine Tip and Casing Heat Transfer132
2.9.3 Vane-Blade Interactions136
2.9.3.1 Cascade Studies137
2.9.4 Deposition and Roughness Effects138
2.9.5 Combustor-Turbine Effects139
2.9.6 Transition-Induced Effects and Modeling141
2.10 Closure143
References144
3.Turbine Film Cooling159
3.1 Introduction159
3.1.1 Fundamentals of Film Cooling159
3.2 Film Cooling on Rotating Turbine Blades162
3.3 Film Cooling on Cascade Vane Simulations169
3.3.1 Introduction169
3.3.2 Effect of Film Cooling171
3.3.3 Effect of Free-Stream Turbulence180
3.4 Film Cooling on Cascade Blade Simulations181
3.4.1 Introduction181
3.4.2 Effect of Film Cooling182
3.4.3 Effect of Free-Stream Turbulence185
3.4.4 Effect of Unsteady Wake186
3.4.5 Combined Effect of Free-Stream Turbulence and Unsteady Wakes193
3.5 Film Cooling on Airfoil Endwalls193
3.5.1 Introduction193
3.5.2 Low-Speed Simulation Experiments193
3.5.3 Engine Condition Experiments200
3.5.4 Near-Endwall Film Cooling201
3.6 Turbine Blade Tip Film Cooling204
3.6.1 Introduction204
3.6.2 Heat-Transfer Coefficient205
3.6.3 Film Effectiveness208
3.7 Leading-Edge Region Film Cooling210
3.7.1 Introduction210
3.7.2 Effect of Coolant-to-Mainstream Blowing Ratio211
3.7.3 Effect of Free-Stream Turbulence213
3.7.4 Effect of Unsteady Wake218
3.7.5 Effect of Coolant-to-Mainstream Density Ratio218
3.7.6 Effect of Film Hole Geometry224
3.7.7 Effect of Leading-Edge Shape225
3.8 Flat-Surface Film Cooling226
3.8.1 Introduction226
3.8.2 Film-Cooled,Heat-Transfer Coefficient227
3.8.2.1 Effect of Blowing Ratio228
3.8.2.2 Effect of Coolant-to-Mainstream Density Ratio229
3.8.2.3 Effect of Mainstream Acceleration231
3.8.2.4 Effect of Hole Geometry233
3.8.3 Film-Cooling Effectiveness239
3.8.3.1 Effect of Blowing Ratio241
3.8.3.2 Effect of Coolant-to-Mainstream Density Ratio242
3.8.3.3 Film Effectiveness Correlations244
3.8.3.4 Effect of Streamwise Curvature and Pressure Gradient250
3.8.3.5 Effect of High Free-Stream Turbulence255
3.8.3.6 Effect of Film Hole Geometry257
3.8.3.7 Effect of Coolant Supply Geometry260
3.8.3.8 Effect of Surface Roughness262
3.8.3.9 Effect of Gap Leakage262
3.8.3.10 Effect of Bulk Flow Pulsations267
3.8.3.11 Full-Coverage Film Cooling267
3.9 Discharge Coefficients of Turbine Cooling Holes269
3.10 Film-Cooling Effects on Aerodynamic Losses272
3.11 New Information from 2000 to 2010276
3.11.1 Film-Cooling-Hole Geometry276
3.11.1.1 Effect of Cooling-Hole Exit Shape and Geometry276
3.11.1.2 Trenching of Holes281
3.11.1.3 Deposition and Blockage Effects on Hole Exits288
3.11.2 Endwall Film Cooling289
3.11.3 Turbine Blade Tip Film Cooling299
3.11.4 Turbine Trailing Edge Film Cooling308
3.11.5 Airfoil Film Cooling310
3.11.5.1 Vane Film Cooling310
3.11.5.2 Blade Film Cooling311
3.11.5.3 Effect of Shocks311
3.11.5.4 Effect of Superposition on Film Effectiveness312
3.11.6 Novel Film-Cooling Designs313
3.12 Closure315
References315
4.Turbine Internal Cooling329
4.1 Jet Impingement Cooling329
4.1.1 Introduction329
4.1.2 Heat-Transfer Enhancement by a Single Jet329
4.1.2.1 Effect of Jet-to-Target-Plate Spacing332
4.1.2.2 Correlation for Single Jet Impingement Heat Transfer333
4.1.2.3 Effectiveness of Impinging Jets334
4.1.2.4 Comparison of Circular to Slot Jets335
4.1.3 Impingement Heat Transfer in the Midchord Region by Jet Array336
4.1.3.1 Jets with Large Jet-to-Jet Spacing337
4.1.3.2 Effect of Wall-to-Jet-Array Spacing337
4.1.3.3 Cross-Flow Effect and Heat-Transfer Correlation339
4.1.3.4 Effect of Initial Cross-Flow345
4.1.3.5 Effect of Cross-Flow Direction on Impingement Heat Transfer346
4.1.3.6 Effect of Coolant Extraction on Impingement Heat Transfer350
4.1.3.7 Effect of Inclined Jets on Heat Transfer354
4.1.4 Impingement Cooling of the Leading Edge355
4.1.4.1 Impingement on a Curved Surface355
4.1.4.2 Impingement Heat Transfer in the Leading Edge356
4.2 Rib-Turbulated Cooling363
4.2.1 Introduction363
4.2.1.1 Typical Test Facility366
4.2.2 Effects of Rib Layouts and Flow Parameters on Ribbed-Channel Heat Transfer368
4.2.2.1 Effect of Rib Spacing on the Ribbed and Adjacent Smooth Sidewalls369
4.2.2.2 Angled Ribs370
4.2.2.3 Effect of Channel Aspect Ratio with Angled Ribs371
4.2.2.4 Comparison of Different Angled Ribs372
4.2.3 Heat-Transfer Coefficient and Friction Factor Correlation375
4.2.4 High-Performance Ribs380
4.2.4.1 V-Shaped Rib380
4.2.4.2 V-Shaped Broken Rib383
4.2.4.3 Wedge- and Delta-Shaped Rib384
4.2.5 Effect of Surface-Heating Condition387
4.2.6 Nonrectangular Cross-Section Channels390
4.2.7 Effect of High Blockage-Ratio Ribs403
4.2.8 Effect of Rib Profile406
4.2.9 Effect of Number of Ribbed Walls413
4.2.10 Effect of a 180° Sharp Turn421
4.2.11 Detailed Heat-Transfer Coefficient Measurements in a Ribbed Channel430
4.2.12 Effect of Film-Cooling Hole on Ribbed-Channel Heat Transfer437
4.3 Pin-Fin Cooling442
4.3.1 Introduction442
4.3.2 Flow and Heat-Transfer Analysis with Single Pin446
4.3.3 Pin Array and Correlation451
4.3.4 Effect of Pin Shape on Heat Transfer459
4.3.5 Effect of Nonuniform Array and Flow Convergence464
4.3.6 Effect of Skewed Pin Array467
4.3.7 Partial Pin Arrangements470
4.3.8 Effect of Turning Flow472
4.3.9 Pin-Fin Cooling with Ejection472
4.3.10 Effect of Missing Pin on Heat-Transfer Coefficient478
4.4 Compound and New Cooling Techniques479
4.4.1 Introduction479
4.4.2 Impingement on Ribbed Walls479
4.4.3 Impingement on Pinned and Dimpled Walls484
4.4.4 Combined Effect of Ribbed Wall with Grooves489
4.4.5 Combined Effect of Ribbed Wall with Pins and Impingement Inlet Conditions491
4.4.6 Combined Effect of Swirl Flow and Ribs495
4.4.7 Impingement Heat Transfer with Perforated Baffles500
4.4.8 Combined Effect of Swirl and Impingement504
4.4.9 Concept of Heat Pipe for Turbine Cooling505
4.4.10 New Cooling Concepts509
4.5 New Information from 2000 to 2010510
4.5.1 Rib Turbulated Cooling510
4.5.2 Impingement Cooling on Rough Surface514
4.5.3 Trailing Edge Cooling517
4.5.4 Dimpled and Pin-Finned Channels518
4.5.5 Combustor Liner Cooling and Effusion Cooling519
4.5.6 Innovative Cooling Approaches and Methods523
References525
5.Turbine Internal Cooling with Rotation537
5.1 Rotational Effects on Cooling537
5.2 Smooth-Wall Coolant Passage538
5.2.1 Effect of Rotation on Flow Field538
5.2.2 Effect of Rotation on Heat Transfer545
5.2.2.1 Effect of Rotation Number546
5.2.2.2 Effect of Density Ratio547
5.2.2.3 Combined Effects of Rotation Number and Density Ratio548
5.2.2.4 Effect of Surface-Heating Condition550
5.2.2.5 Effect of Rotation Number and Wall-Heating Condition554
5.3 Heat Transfer in a Rib-Turbulated Rotating Coolant Passage556
5.3.1 Effect of Rotation on Rib-Turbulated Flow556
5.3.2 Effect of Rotation on Heat Transfer in Channels with 90° Ribs559
5.3.2.1 Effect of Rotation Number560
5.3.2.2 Effect of Wall-Heating Condition563
5.3.3 Effect of Rotation on Heat Transfer for Channels with Angled (Skewed) Ribs565
5.3.3.1 Effect of Angled Ribs and Heating Condition567
5.3.3.2 Comparison of Orthogonal and Angled Ribs572
5.4 Effect of Channel Orientation with Respect to the Rotation Direction on Both Smooth and Ribbed Channels572
5.4.1 Effect of Rotation Number572
5.4.2 Effect of Model Orientation and Wall-Heating Condition574
5.5 Effect of Channel Cross Section on Rotating Heat Transfer582
5.5.1 Triangular Cross Section582
5.5.2 Rectangular Channel585
5.5.3 Circular Cross Section587
5.5.4 Two-Pass Triangular Duct588
5.6 Different Proposed Correlation to Relate the Heat Transfer with Rotational Effects596
5.7 Heat-Mass-Transfer Analogy and Detail Measurements603
5.8 Rotation Effects on Smooth-Wall Impingement Cooling604
5.8.1 Rotation Effects on Leading-Edge Impingement Cooling604
5.8.2 Rotation Effect on Midchord Impingement Cooling613
5.8.3 Effect of Film-Cooling Hole618
5.9 Rotational Effects on Rib-Turbulated Wall Impingement Cooling619
5.10 New Information from 2000 to 2010623
5.10.1 Heat Transfer in Rotating Triangular Cooling Channels625
5.10.2 Heat Transfer in Rotating Wedge-Shaped Cooling Channels633
5.10.3 Effect of Aspect Ratio and Rib Configurations on Rotating Channel Heat Transfer643
5.10.4 Effect of High Rotation Number and Entrance Geometry on Rectangular Channel Heat Transfer666
References683
6.Experimental Methods689
6.1 Introduction689
6.2 Heat-Transfer Measurement Techniques689
6.2.1 Introduction689
6.2.2 Heat Flux Gages690
6.2.3 Thin-Foil Heaters with Thermocouples693
6.2.4 Copper Plate Heaters with Thermocouples697
6.2.5 Transient Technique698
6.3 Mass-Transfer Analogy Techniques699
6.3.1 Introduction699
6.3.2 Naphthalene Sublimation Technique699
6.3.3 Foreign-Gas Concentration Sampling Technique703
6.3.4 Swollen-Polymer Technique705
6.3.5 Ammonia-Diazo Technique706
6.3.6 Pressure-Sensitive Paint Techniques707
6.3.7 Thermographic Phosphors710
6.4 Liquid Crystal Thermography713
6.4.1 Steady-State Yellow-Band Tracking Technique713
6.4.2 Steady-State HSI Technique714
6.4.3 Transient HSI Technique717
6.4.4 Transient Single-Color Capturing Technique719
6.5 Flow and Thermal Field Measurement Techniques726
6.5.1 Introduction726
6.5.2 Five-Hole Probe/Thermocouples726
6.5.3 Hot-Wire/Cold-Wire Anemometry728
6.5.4 Laser Doppler Velocimetry729
6.5.5 Particle Image Velocimetry731
6.5.6 Laser Holographic Interferometry734
6.5.7 Surface Visualization734
6.6 New Information from 2000 to 2010739
6.6.1 Transient Thin-Film Heat Flux Gages739
6.6.2 Advanced Liquid Crystal Thermography743
6.6.3 Infrared Thermography746
6.6.4 Pressure-Sensitive Paint749
6.6.5 Temperature-Sensitive Paint755
6.6.6 Flow and Thermal Field Measurements759
6.7 Closure761
References761
7.Numerical Modeling771
7.1 Governing Equations and Turbulence Models771
7.1.1 Introduction771
7.1.2 Governing Equations772
7.1.3 Turbulence Models773
7.1.3.1 Standard κ-ε Model773
7.1.3.2 Low-Reκ-ε Model774
7.1.3.3 Two-Layerκ-ε Model775
7.1.3.4 κ-ω Model775
7.1.3.5 Baldwin-Lomax Model776
7.1.3.6 Second-Moment Closure Model777
7.1.3.7 Algebraic Closure Model777
7.2 Numerical Prediction of Turbine Heat Transfer779
7.2.1 Introduction779
7.2.2 Prediction of Turbine Blade/Vane Heat Transfer779
7.2.3 Prediction of the Endwall Heat Transfer785
7.2.4 Prediction of Blade Tip Heat Transfer787
7.3 Numerical Prediction of Turbine Film Cooling789
7.3.1 Introduction789
7.3.2 Prediction of Flat-Surface Film Cooling791
7.3.3 Prediction of Leading-Edge Film Cooling796
7.3.4 Prediction of Turbine Blade Film Cooling798
7.4 Numerical Prediction of Turbine Internal Cooling799
7.4.1 Introduction799
7.4.2 Effect of Rotation799
7.4.3 Effect of 180° Turn803
7.4.4 Effect of Transverse Ribs809
7.4.5 Effect of Angled Ribs809
7.4.6 Effect of Rotation on Channel Shapes815
7.4.7 Effect of Coolant Extraction818
7.5 New Information from 2000 to 2010820
7.5.1 CFD for Turbine Film Cooling820
7.5.2 CFD for Turbine Internal Cooling823
7.5.3 CFD for Conjugate Heat Transfer and Film Cooling825
7.5.4 CFD for Turbine Heat Transfer829
References830
8.Final Remarks841
8.1 Turbine Heat Transfer and Film Cooling841
8.2 Turbine Internal Cooling with Rotation841
8.3 Turbine Edge Heat Transfer and Cooling842
8.4 New Information from 2000 to 2010842
8.5 Closure843
Index845
热门推荐
- 1518343.html
- 1243211.html
- 780189.html
- 649987.html
- 608196.html
- 529771.html
- 105928.html
- 314176.html
- 2462085.html
- 290635.html
- http://www.ickdjs.cc/book_2561049.html
- http://www.ickdjs.cc/book_1142842.html
- http://www.ickdjs.cc/book_3264415.html
- http://www.ickdjs.cc/book_1866226.html
- http://www.ickdjs.cc/book_147764.html
- http://www.ickdjs.cc/book_1795975.html
- http://www.ickdjs.cc/book_2804315.html
- http://www.ickdjs.cc/book_3095260.html
- http://www.ickdjs.cc/book_1763554.html
- http://www.ickdjs.cc/book_1640298.html